Helicopter structure, aerodynamics and control
How does a helicopter fly? On this page we show you how a helicopter is constructed and how it flies. These are important requirements before your first trial flight. The information is divided into different sections.
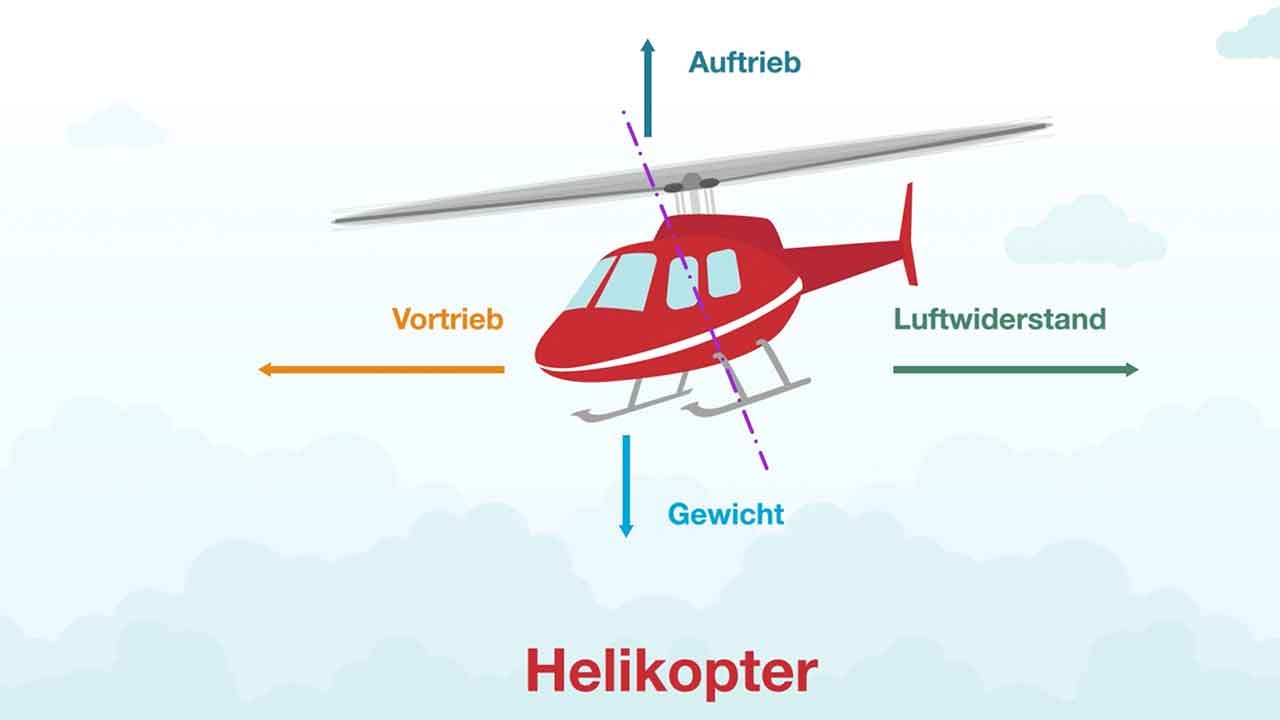

How can I fly a helicopter myself
1. Helicopter rotor system



Articulated rotors
The rotor blades of the first helicopters were rigidly connected to the rotor shaft. This led to an uncontrollable rolling motion in forward flight, as the leading rotor blade provided more lift than the one in the rear due to the higher airflow speed. This repeatedly led to problems and even crashes. The first person to introduce flapping and swivel joints was Juan de la Cierva in his autogiros.
Today’s modern rotor systems can be divided into the following categories:
- articulated rotors
- jointless rotors
- bearingless rotors
The articulated rotor systems have mechanical flapping and swivel joints, as well as a bearing for adjusting the pitch angle of the rotor blade (Fig. 1). These joints are very maintenance-intensive and therefore expensive to maintain.
jointless rotors
In the jointless rotor system, the mechanical flapping and swivel joints are replaced by correspondingly flexible materials at the blade root, which allow the flapping and swiveling movement (Fig. 2). This only became possible with the introduction of plastics. The BO-105 was the first helicopter with such a rotor system.
bearingless rotors
The bearingless rotor has no mechanical flapping or swivel joints and the bearing for adjusting the pitch angle is also replaced by an elastomer bearing. Since no conventional mechanical bearings are used, maintenance can be significantly reduced. Very large forces act on the rotor blade root, which is why the bearingless rotor with elastomer bearing is only suitable for smaller helicopters. This system was implemented by Aerospatial with the Spheriflex rotor.
The semi-rigid system can also be classified in the group of articulated rotor systems (Fig. 3). This method is only used in helicopters with two rotor blades. The two rotor blades are connected to the rotor mast via a type of rocker, which allows the flapping movement. Swivel joints are not required in this system.
2. Helicopter Aerodynamics




Aerodynamic Basics
Before we can get into helicopter aerodynamics, we should know some basic aerodynamic principles.
In order for aircraft that are "heavier than air" to take off from the ground, an upward force must act that is at least as great as the weight of the aircraft. This force is called lift and is generated by the wings.
drive
The wings have a specific shape in cross-section, the profile. There are a variety of different profile types, depending on the flight characteristics an aircraft should achieve.
If a wing now moves forward, the profile divides the airflow into a lower and an upper part (Fig. 1).
suction effect
Since the air is displaced by the curvature around the profile, it has to travel a "longer distance", which increases the flow speed. According to the law of fluid dynamics (Bernoulli equation), the increase in speed leads to a reduction in pressure. A "suction" is created on the surface of the wing (Fig. 2). Since the upper and lower sides of the profile have a different curvature, a different "suction" is also created.
air resistance
With a fully symmetrical profile (a semi-symmetrical one is shown here), the negative pressure on the upper side of the wing is exactly the same as on the lower side.
These purely aerodynamic forces are not enough to make an aircraft fly. A wing must be slightly tilted in the airflow, which deflects the air downwards, resulting in excess pressure on the underside of the wing, which increases the overall lift (Fig. 3).
angle of attack / vortex
This angle of attack also causes an increase in the negative pressure on the upper side, as the air has to travel an even further distance and is therefore accelerated more.
However, the angle of the wing also increases air resistance, which must be compensated for with greater power for propulsion.
Basically, it can be said that the faster the aircraft moves forward, the greater the lift. At the same time, however, air resistance also increases. For this reason, aircraft that fly slowly have thick profiles, while very fast aircraft only need slim profiles to generate lift.
However, the angle of attack and the speed cannot be increased arbitrarily because the air flow on the upper side can break off. This means that the flow no longer flows along the profile, but forms vortices (Fig. 4).
First, the vortices form at the trailing edge. If the angle of attack is increased further, more and more vortices form towards the leading edge until the lift is no longer sufficient to keep the aircraft in the air. This flight condition is called a stall and occurs primarily when the aircraft is flying too slowly.
As soon as the airflow flows cleanly along the profile again, the necessary lift is present again and the aircraft flies again.
Differences between helicopter and airplane



Airplane
Helicopters are fundamentally different from fixed-wing aircraft. Although aerodynamic forces are also at work in helicopters, they are much more difficult to calculate and explain than in airplanes. This is mainly because the rotating rotor creates additional forces that are not present in fixed-wing aircraft.
In an aircraft with wings, the situation is fairly clear. Propulsion is provided by either a propeller or a jet engine (except in a glider). Lift is generated by the wings and the whole thing is controlled by flaps, rudders and tail units (Fig. 5).
helicopter
The situation is different with a helicopter. The rotating rotor blades generate lift, similar to a wing, and accelerate the air from top to bottom. This happens by simultaneously increasing the pitch angle (angle between the rotor blade chord and the helicopter's longitudinal axis) of all the rotor blades, and thus also the angle of attack. This is known as collective blade adjustment. This "blows" the air downwards, similar to a fan, the overall lift is increased and the helicopter begins to climb. In order for this vehicle to move forward, "only" the rotor plane needs to be tilted forwards so that the air flow is "blown" slightly backwards through the rotor (Fig. 6).
helicopter control
The helicopter is controlled using the same principle. The rotor plane is tilted in the direction the helicopter is supposed to fly. This sounds very simple, but in reality it is a very complex aerodynamic process (we will talk about this later). In fact, a physical law (of Newton) states that an action causes a reaction. This causes the fuselage of the helicopter to want to rotate in the opposite direction to the rotation of the rotor. To prevent this, most helicopters have a vertically rotating rotor, the tail rotor, which compensates for this torque (Fig. 7). With this tail rotor, the helicopter can be controlled around the vertical axis in hovering flight.
In designs with two counter-rotating main rotors, no torque is generated on the fuselage, or rather the torques of the two rotors cancel each other out.
The helicopter hovering



boost
In contrast to fixed-wing aircraft, helicopters can fly forwards, sideways and backwards, but can also remain stationary in the air. This is possible because the main rotor blades are always exposed to air flow due to their rotation, thus providing the necessary lift. A fixed-wing aircraft only generates lift when a sufficiently high forward speed is reached.
For the sake of simplicity, in hovering flight we will consider the rotor as a disk and not examine the conditions on the individual rotor blade. This is possible because the aerodynamic forces are distributed somewhat symmetrically across the entire rotor disk.
In order for a helicopter to stay in the air, its lift must be exactly equal to its weight (Fig. 8).
angle of attack
If the angle of attack of all rotor blades is increased simultaneously using the collective blade adjustment, the air flow from top to bottom through the rotor disk increases, the lift increases and the helicopter begins to climb on the spot (Fig. 9).
If the angle of attack is reduced, the total lift decreases and the helicopter begins to descend (Fig. 10).


rotation of the main rotor
The rotation of the main rotor creates a moment that causes the fuselage to rotate in the opposite direction to the rotation of the main rotor. This unwanted rotation is corrected by the vertical tail rotor (Fig. 11). The greater the power of the main rotor, the greater the torque, and the more the tail rotor has to do to correct the torque.
Since the tail rotor produces a certain horizontal thrust, the helicopter has a tendency to move in the corresponding direction. The direction depends on the direction of rotation of the main rotor.
Stationary hovering by lateral displacement
This lateral displacement must in turn be corrected with the main rotor. The air flow, also called downwash, is directed slightly against the direction of displacement, which keeps the helicopter in a stationary hover.


hovering outside ground effect
In many helicopters, the forces of the main and tail rotors do not act in the same horizontal plane. For this reason, the helicopter may not be horizontal when hovering, but may be slightly banked. Whether the bank is to the left or right depends primarily on the direction of rotation of the main rotor (Fig. 12).
Hovering generally requires more power than forward flight. Air density plays a key role in performance. The denser the air, the less power the engine needs and the more weight the helicopter can carry. As air density decreases with increasing altitude, the weight of the helicopter must be reduced in order to be able to keep it in hovering flight. In general, the higher the outside temperature and the higher the altitude, the lower the performance of the helicopter.
Downwash also has an influence on performance. If the air flow can flow away unhindered, this condition is called hover out of ground effect (OGE) (Fig. 13).
Air cushions - for stationary hovering
If the helicopter hovers close to the ground, this is called hovering in ground effect (IGE). The downwash, which must be diverted to the side, creates a kind of air cushion (Fig. 14). This means that the helicopter needs less power for stationary hovering.
The higher the helicopter hovers above the ground, the smaller the influence of the ground effect. At a hovering height of about 1.5x the rotor diameter, there is no longer any ground effect. The nature of the ground and especially the slope of the terrain also have a major influence on the ground effect. The more the ground is sloped, the better the downwash can flow away and the smaller the ground effect.
The helicopter in forward flight


forward flight
The greatest advantage of helicopters is that they can both hover and fly forwards. The transition from hovering to forward flight is called the transition and is an extremely complicated process both aerodynamically and mechanically. For the sake of simplicity, we will consider the rotor as a disk and not the aerodynamic conditions on the individual rotor blade.
As already mentioned, the air is accelerated from top to bottom by the rotor during hovering (Fig. 15). In order for the helicopter to enter forward flight, the entire rotor disk must be tilted forward.
inclination
Due to the forward tilt, the air is no longer accelerated vertically downwards, but backwards (Fig. 16). This causes the helicopter to start moving forwards. However, since the lift no longer acts vertically upwards, the pilot must increase the power slightly during the takeoff phase in order to achieve the correct ratio between lift and weight.



flow velocities
The rotation of the rotor creates different airflow speeds on the rotor blades during forward flight. The rotor blade that moves forward in the direction of flight is called the leading blade, and the one that moves backward is called the retreating blade (Fig. 17).
speed of the advancing blade
The approach speed depends on the forward speed, the rotor speed and the rotor diameter. If we assume that the helicopter is moving forward at a speed of 200 km/h and has a blade tip speed of 750 km/h, the following conditions arise at the rotor:
The leading blade reaches an effective speed of 950 km/h (750 + 200) at the blade tip. This speed is already very close to the speed of sound. At the blade root, the flow is still over 200 km/h (Fig. 18).
speed of the returning blade
The receding blade is only approached by airflow at a speed of 550 km/h at the blade tip (750 - 200). The airflow speed decreases the closer you are to the center of rotation. In the area of the blade root, the blade can even be approached from behind and therefore no longer provides lift in this area (Fig. 19).
As is well known, lift depends on the airflow velocity and the angle of attack (as well as the type of profile). In order to achieve a fairly constant lift ratio across the entire rotor disk, the angle of attack must be constantly changed as the blade rotates, since the airflow velocity also changes constantly. This adjustment of the angle of attack is referred to as cyclic blade adjustment.
The limits of forward flight for today's helicopters are around 400 km/h. Above this speed, large parts of the leading blade would be in the supersonic range and a large part of the trailing blade would be in stall. There is no wing profile that could cover such a large speed range.
The autorotation
In order to ensure the airflow to the rotor blade, the rotor must always be driven. But what happens if the drive fails - for whatever reason?
Since the rotor blades have a relatively large angle of attack during forward flight due to the collective blade adjustment and therefore also produce a correspondingly large air resistance, the speed of the rotor drops rapidly without propulsion. This of course also means that the necessary lift is lost and the helicopter crashes within a short time.
Fortunately, that sounds much more dramatic than it actually is. What gliding is for a fixed-wing aircraft, autorotation is for a helicopter. If the helicopter's engine fails during flight, the pilot will immediately reduce the collective blade pitch and the helicopter will begin to descend. At the same time, the air resistance on the rotor blades is significantly reduced due to the smaller angle of attack.
aerodynamic conditions
As we can see in Fig. 20, the air no longer flows through the rotor from top to bottom, but from bottom to top. Due to the aerodynamic conditions, which we will look at in more detail, the rotor speed can be kept constant in this state. In order to explain the processes in autorotation, we cannot consider the rotor as a disk as before, but must examine the conditions on the individual rotor blade. And to do this, we first look at the state in powered forward flight (Fig. 21).


vertical descent
In a flight profile, the lift always acts perpendicular to the incoming flow and the air resistance in the same plane as the incoming flow. Since the incoming flow in a helicopter consists of a horizontal component (rotation of the rotor) and a vertical component (air flow from above or below), we speak of a relative incoming flow in the case of the rotor blade. Since the rotor blade moves at a higher speed towards the outside, but the vertical component remains more or less constant, the relative incoming flow changes constantly over the entire length of the rotor blade. For this reason, Fig. 21 only applies to a small area of the rotor blade. The angle of attack (angle between the profile chord and the relative incoming flow) also changes over the length of the rotor blade, and the angle of attack decreases towards the outside.
In the autoration, the rotor can be divided into three areas. For the sake of simplicity, we first look at the vertical autoration, which means that the helicopter is in vertical descent (Fig. 22).
Vertical autorotation
In vertical autorotation, the areas are distributed symmetrically across the rotor disk. Only the driving area is responsible for the rotation of the rotor. Near the center, the flow velocity is so low that the rotor blades are in a stall.
To explain how these areas come about, we need to examine the aerodynamic conditions on the individual rotor blade in more detail.
In the driving area, the total aerodynamic forces are in front of the rotation axis of the rotor. This results in a force that drives the rotor (Fig. 23).
If the aerodynamic forces lie exactly on the axis of rotation, the rotation speed remains constant (Fig. 24).
In the braking part, the total forces are behind the axis of rotation, which means that the rotor is braked (Fig. 25).
balance
All helicopters are designed to achieve a balance between the propulsion and braking parts. This balance must be maintained from vertical descent up to a certain forward speed. Some helicopters are limited in their maximum forward speed during autorotation. This is because the propulsion part shifts as the speed increases (Fig. 26). This shift always occurs in the direction of the returning blade.
If the forward speed is increased again in this situation, the driving area shifts further to the right, which ultimately leads to the braking part becoming larger than the driving part and thus the rotor speed can no longer be kept constant.
As a rule, an autorotation is always flown at a certain forward speed. To ensure a safe landing, this speed must be reduced as much as possible. This is achieved with the so-called flare. Just above the ground, the pilot raises the nose, which reduces the sink rate and reduces the speed. This braking allows the rotor to absorb even more energy (the speed is increased) and the helicopter can perform an almost normal landing. This sounds very simple, but it is a demanding maneuver for the pilot.





The Vortex
air flow hover
During stable hovering, as already described, the air is accelerated from top to bottom by the rotor. The air flow rate is not constant over the length of the rotor blade (Fig. 27).
vortex ring state
The vortex or swirl ring condition occurs in a helicopter when it is hovering or slowly flying forward with a high rate of descent. The rate of descent must be in a range of more than 500 ft/min (2.5 m/sec) and the forward speed must be below the so-called transition zone. In addition, the rotor must be driven by the engine. These conditions are particularly present during a steep landing approach with a tailwind.
The sinking speed creates an air flow which counteracts the downwash (Fig. 28).
Vortex
As a result, the air in the inner area of the rotor plane is accelerated from top to bottom, but is immediately transported back up again by the air flow from below (Fig. 29).



vortex ring state
The air is sucked in again from above and this creates a closed system, the vortex or vortex ring state (Fig. 30). In this state the helicopter begins to sink even more, even if the power is increased. The helicopter is still controllable, but strong vibrations sometimes occur.
The vortex can basically be ended in two ways: The first option is to go into forward flight. Since the downwash is diverted backwards in forward flight, the rotor can be supplied with "new air" from above. This variant must be used if the vortex occurs close to the ground, for example during a landing approach.
As a second possibility, you can switch to autoration, whereby the air flow from top to bottom is missing and the rotor is only flowed through from bottom to top (as in a normal autoration).
In any case, the pilot must react very quickly when the vortex occurs, because the enormous rate of descent may mean that the flight altitude is too low to end the vortex.

3. Controlling a helicopter
The Basics of Control
A helicopter is controlled with three different controls. The control stick, also called cyclic, is used in hovering flight to move the helicopter left, right, forward or backward. In forward flight, the cyclic initiates turns or climbing and descending. The pedals can be used to turn the helicopter around the vertical axis in place, and with the collective the pilot controls the climbing and descending in hovering flight, and together with the cyclic the speed in forward flight (Fig. 1).

setting angle of rotor blades
In every helicopter, the control stick is operated with the right hand and the collective with the left. The feet are used to control the tail rotor via the pedals. Due to the instability of a helicopter, the cyclic must not be let go during flight unless the helicopter is equipped with an automatic stabilization system.
The control elements (cyclic, collective and pedals) are used to mechanically adjust the setting angle of the main rotor and tail rotor blades. The setting angle is the angle between a defined line on the helicopter and the setting of the rotor blade profile. The angle of attack, on the other hand, is the angle between the rotor blade profile and the incoming air. This means that when the setting angle is adjusted via the control, the angle of attack and thus the lift is also changed.
As soon as the pilot pulls up on the collective, the setting angle (and thus the angle of attack) of all rotor blades is increased by the same amount. This increases the overall lift and the helicopter begins to climb. The setting angle decreases accordingly when the pilot pushes the collective downwards. (Fig. 2)
If the Cyclic is pushed forward, the setting angle of the rotor blades is continuously changed during one rotation. Due to the different lift, the rotor blade plane is tilted forward. Of course, the plane tilts backwards as soon as the pilot pulls the Cyclic backwards. (Fig. 3)
The same as described above happens when the pilot pushes the Cyclic either to the right or to the left. (Fig. 4)
The pedals are used to adjust the pitch of all tail rotor blades by the same amount (similar to the collective blade adjustment on the main rotor). This increases or decreases the tail rotor thrust. (Fig. 5)
There is just one small catch to the whole thing with the controls. As soon as the pilot changes something on one of the three controls, he has to correct the other two as well. As we know, the pilot has to pull on the collective if he wants to climb a little while hovering. This means that the torque increases due to the larger angle of attack of the rotor blades (and thus increased air resistance). This causes the helicopter to start rotating around its vertical axis, which can only be prevented by correcting the tail rotor. Since the tail rotor now provides greater lateral thrust, the lateral displacement of the helicopter is also increased, which in turn can only be compensated for by correcting the cyclic. You might think that the helicopter is now in a stable hover. Unfortunately, that is not the case!! Due to external influences (especially wind) and the inconsistent turbulence around the helicopter, the pilot always has to correct all the controls in order to maintain a stable hover. The big difficulty is coordinating all these small corrections correctly. Only when a student pilot no longer has to study which control to use to correct can he keep the helicopter in a more or less stable hover. And it takes some time (and a few drops of sweat) to train this automatic behavior.




The technology of control
The control of a helicopter is ensured via control rods, bellcranks and control cables, from the control elements to the swashplate (or to the tail rotor) (Fig. 6).
swashplate
The most important element of a helicopter's control system is the swashplate. It is located on the rotor axis, directly under the main rotor, and is responsible for transmitting the control impulses to the individual rotor blades. The swashplate basically consists of two parts, the fixed part and the rotating part. The fixed or lower part is connected to the control stick via control rods and bellcranks, and the rotating or upper part is connected to each individual rotor blade. If the swashplate is tilted forwards or to the side using the control stick, the setting angle of each rotor blade changes during one revolution (cyclic blade adjustment). If the pilot pulls up on the collective, the swashplate as a whole moves up and the setting angle of all rotor blades is increased simultaneously (collective blade adjustment). This principle works regardless of the number of rotor blades in the helicopter (Fig. 7).
In smaller helicopters, the control is usually supported by hydraulic servo motors to reduce the effort required by the pilot. In medium and large helicopters, the forces on the swashplate are so great that purely mechanical control (only with control rods and bellcranks) is no longer possible and control can only be ensured with hydraulic support. The control of the tail rotor works in basically the same way. The main difference is that only the collective blade adjustment is required. The pedals in the cockpit are connected to the so-called spider via control rods, bellcranks and control cables. The spider transmits the control impulses to the individual tail rotor blades, similar to the swashplate on the main rotor. Here too, the larger the helicopter, the greater the control forces on the tail rotor and the more the control must be supported with hydraulic aids (Fig. 8).



NOTARY
The NOTAR Principle
The NOTAR (NO TAil Rotor) is a helicopter with a main rotor but no tail rotor. This process was developed and patented by McDonnell Douglas (MD-Helicopters). The great advantage of this system is that there is no danger from the rotating tail rotor. The noise level of the helicopter is also significantly reduced. In a conventional helicopter, most of the noise is caused by the tail rotor.
The principle of the NOTAR is actually quite simple. Part of the main rotor downwash is directed into the tail boom and further compressed there by a fan. The air now flows through the tail boom and escapes at the end through swivel nozzles. These nozzles are controlled by the pedals and allow the torque to be balanced and the helicopter to rotate around its vertical axis (Fig. 9).
This effect is supported by the special shape and additional outlet nozzles along the tail boom. The air only flows out on one side, which deflects the downwash to the side and accelerates it. This creates a lateral aerodynamic lift (Fig. 10). With this little trick, you can achieve the effect of a normal wing profile even with a round body.
This system is mainly used for control during hovering and slow forward flight. During fast forward flight, the helicopter is controlled via vertically arranged rudders in the vertical axis.
The control around the vertical axis is also fully functional in autorotation, since the fan, which generates the necessary air flow in the tail boom, is mechanically connected to the main gearbox.
Courtesy of hubschrauber.li


